About project
Recent experiments with microscopic systems exhibiting signatures of quantum coherence and entaglement have spurred interest in exploiting those newly developed capabilities in practical applications. The emerging domain of quantum-enhanced technologies has the potential to transform many areas of science and engineering.
This potential is visible most clearly in the field of information technologies. While quantum mechanics plays the key role in defining fundamental limits of performance of information systems, it also offers the way to push state-of-the-art in information technologies significantly forward. One example is quantum computation, where interference between different processing paths can drive the computer to a sought solution much faster than it is possible using best known classical algorithms. The other is quantum cryptography using quantum systems that can be employed to generate a cryptographic key whose security is guaranteed by fundamental physical laws.
Since photons are excellent carriers of information over long distances, many of the most promising physical implementations for quantum-enhanced technologies are based on photonic systems.
The purpose of the present project is to address problems at the current forefront of the quantum-enhanced technologies. The proposed research should open up new ways of exploiting quantum phenomena in information technologies. Our goals are grouped into three Themes:
Theme 1: Light-matter interface
Currently, manipulating photonic states is possible only within the operations performed by optical elements that interact with photons as they pass through them. The light-matter interface would add to that one important capability - storing quantum superpositions by mapping them onto atomic systems (and retrieving at a given moment).
In most cases, generating particular quantum states of light necessary to implement quantum-enhanced protocols is performed by the process of parametric down-conversion. However, using this technique limits the possibility of achieving higher photon number to very few. In fact, the probability of the situation when all the parallel sources employed to generate photons produce them simultaneously decreases exponentially with number of photons required.
The difficulties can be circumvented with the help of atomic-vapour based photon sources. Using weakly driven Raman transition in polarized atomic sample it is possible to engineer a situation when one atom in the sample is excited and one photon is scattered (called the heralding photon), providing the information about atomic excitation. The excitation can be stored for an extended time and then converted into a single photon, useful in a particular experiment. Moreover, multiple independent excitations can be generated in the same ensemble and distinguished by properties of their heralding photons. This feature allows to overcome the exponential scaling of preparation time of a number of photons.
Theme 2: Quantum-enhanced protocols in realistic environments
Several quantum protocols, for example methods of quantum computation, quantum cryptography, and quantum enhanced parameter estimation are theoretically well-understood. However, many models do not include phenomena that inevitably accompany experimental implementations of the protocol. The realizations are usually affected by decoherence, uncontrolled state evolution and loss.
The goal of this part of the Project is to develop quantum-enhanced protocols robust to certain decoherence mechanisms and provide proof-of-principle demonstrations of their feasibility.
In general, a quantum information processing task consists of three stages - a preparation of quantum state, unitary evolution and quantum measurement. Since preparation and measurement stages are usually performed relatively quickly, they are less affected by external disturbance in comparison to the processing stage.
In more detail, problems addressed in theme 2 include:
- Research on phase measurements in the presence of losses in order to bridge the gap between current theory and experimental capabilities.
- Sending photon polarization states over long optical fibers, which demonstrate fluctuating birefringence leading to polarization mode dispersion and decoherence. This obstacle can be overcome by encoding information with help of entangled states.
- Photon loss, which could have disastrous effect on quantum protocols involving only a few photons. Although cloning quantum states is impossible, other method of dealing with loss has been proposed, and that is a use of quantum repeaters. Experimental implementation of those would utilize the light-matter interfaces investigated in Theme 1.
Theme 3: Simulations of light-matter interaction
This part of the project involves creating theoretical models of some processes that quantum-enhanced technologies are based on. The processes in question, so far theoretically described in an insufficient way, include parametric down-conversion, four-wave mixing and stimulated Raman scattering.
Theoretical treatment of those nonlinear processes is excessively simplified, therefore it often produces results in which properties of non-classical radiation are compromised. The need for more accurate models shows itself in experimentally observed features that are not predicted in theory. An example of application of quantum field theories in new environments is a soliton, theoretically described as a solution to nonlinear Shroedinger equation, which has recently been confirmed experimentally (as a nonlinear phenomenon related to light propagation through an optical fiber). Quantum field theory effects can prove important in fiber-optic communications operating at intensities close to quantum limits.
Another goal is to investigate light-matter interactions in the context of decoherence mechanisms. Together with findings of Theme 2, those can result in important proof-of-principle demonstrations.
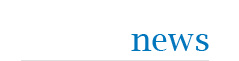